to the project website of [e-Speicher]3 – applied solvation research in the Ruhr area. We seek to fasten electrochemical energy storage with three-dimensional architectures of materials with designed nanoscaled water channels.
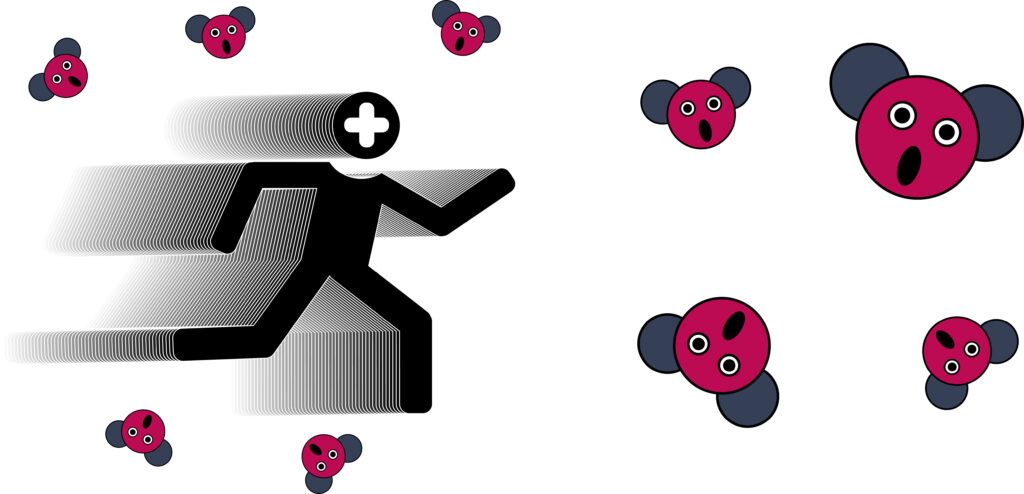
[e-Speicher]³ is …
… a research project striving to provide the physicochemical understanding needed for tunable sustainable storage of electrical energy based on synergistic material and electrode design
During the last decade, nanomaterials have been developed for a variety of electrochemical energy storage technologies, such as batteries and supercapacitors, achieving increased energy and power densities. However, often excellent performance parameters are only obtained in laboratory scale tests and not in technical devices.
For example, capacitances are given in Farad per gram of active nanomaterial even though this mass-specific value decreases for higher mass loadings of the nanomaterial. If thicker films of nanomaterials are used in energy storage devices, e.g., electric conductivity and transport of active components in the interfacing liquid electrolyte may be affected.
So, high-performance nanomaterials are processed to electrodes which are used in terminal devices. Though, how well a device performs significantly depends on how the active film of the electrode is assembled. How thick is this film? How densely are nanoparticles packed? How large are the contact areas of neighbouring nanoparticles? How many electrolyte-filled void spaces are there, and of which size they are? etc. To this end, specifically adjusted three-dimensional architectures of tailored nanomaterials must be developed. Besides suitable, scalable fabrication processes, understanding of nanomaterial and electrode shape effects is needed to improve electrochemical energy storage technologies.
… analyzing properties of nano-building-blocks and buildings made of them
The development of electrochemical energy storage devices such as e-bike batteries concerns many different levels. So to speak, a battery pack resembles an estate of terraced houses, with a battery being one terraced house consisting of various battery cell components: a roof, walls, etc. How many people comfortably can live in the house and if it soon will require renovations obviously depends not only on the quality of the bricks. A lot more matters: from the way the bricks are connected, to the architecture, and even the people living in the house.
The same holds for an electrochemical energy storage electrode. The nanoparticles constituting a storage-active film may have intrinsic electrochemical properties which, however, are not simply summed up when billions of them are built up to an electrode. To research such ensemble effects of nanoparticles influencing each other, we employ customized electroanalytical methods to characterize complete electrodes as well as nano-impact approaches to gain statistical information on electrochemical properties of single nanoparticles. To this end, we can employ the knowledge on brick quality and shell construction to improve electrode architectures. Read more
… elucidating storage mechanisms in nanoscaled water channels
To electrochemically store energy, changes at the active storage material are triggered by applying a voltage. Under conditions where the material transitions back to its original state, electric current can be (re-)utilized to power applications. The respective changes depend on the interactions of a solid storage material with components of a surrounding liquid – the interfacing electrolyte. Thus, we need to understand how materials alter in course of energy storage and supply, as well as effects of shape and structure to systematically improve energy storage properties.
[e-Speicher]3 investigates active electrode architectures employing nanoparticles which are pervaded by many narrow channels. In contrast to a massive material block, such nanomaterials provide a large interface to the liquid electrolyte. Therefore, the material can fast contribute to electrochemical energy storage to a large extent. For the process of storage-related material changes several possibilities are plausible according to the current state of knowledge.
One feasible explanation is based on ions dissolved out of the solid-state material during storage and re-integration into the solid material when electric current is supplied. If such a mechanism is observed, the shape of the nanomaterial can be adjusted to ensure that many dissolved ions remain close to the material surface. For example, nanoparticles can be assembled with void spaces between them only accessible through narrow electrolyte filled inlets.
An alternative storage mechanism is based on changes of the solid-state material which are not accompanied by ions being disintegrated from the compound. These alterations require re-organization of solvent molecules and conducting salt components of the electrolyte at the liquid/solid interface to compensate the energy-storing material changes. Accordingly, solvation properties in the narrow sub-nanometre channels of the nanoparticles change. Thus, the nanoparticle shape and electrode architecture should be adjusted to allow efficient exchange of electrolyte components between the channels and particle surrounding regions.
… developing sustainable methods to build three-dimensional architectures
Knowing which electrode architectures serve the function is only a part of the solution. The next step requires new fabrication approaches to provide such architectures in a scalable and sustainable manner. Electrodeposition methods entail the inherent conceptional advantage to ensure electrical contact of deposited materials and can provide for a wide range of electrode architectures and material classes. One approach we are exploring takes advantage of usually unwelcome side reactions during electrodeposition from aqueous solutions. Gas bubbles evolving due to electrochemical water splitting at elevated voltages are used as dynamic templates to obtain 3-dimensional electrode morphologies. Read more
Learn about Electrochemistry
The graduate programmes (Master/PhD) in Chemistry at the RUB offer the courses Electrochemistry: From Fundamentals to Applications I (winter term) and II (summer term). Julia teaches the parts on Electrochemical Impedance Spectroscopy (EIS).
Although frequency dependent electrochemical measurements provide access to intriguing information about electrochemical kinetics and transport (sub-)processes, getting started with EIS can be challenging. Therefore, we have created a series of videos to make the method accessible regardless of prior knowledge. We provide detailed explanations of the key terms and experimental examples to help viewers understand the concepts, fill in gaps, and promote connections within their knowledge maps. In this way, we hope to prepare our students and interested electrochemistry enthusiasts for more advanced topics in EIS, such as those covered in the courses at the RUB.
We also offer in-depth and focal point practicals, as well as bachelor and master theses in analytical/physical electrochemistry related to energy storage and conversion. Please, contact Julia if you are interested in doing research with our team.
Check out related publications
S. Reinke, V. Khamitsevich, O. Röth and J. Linnemann “Assessment of the Physicochemical Meaning of the Ohmic Series Resistance Observed for High Frequencies in Electrochemical Impedance Spectra” International Workshop on Impedance Spectroscopy (IWIS), 2023, 45, DOI: 10.1109/IWIS61214.2023.10302764.
A. Rabe, M. Jaugstetter, F. Hiege, N. Cosanne, K. F. Ortega, J. Linnemann, K. Tschulik, M. Behrens “Tailoring Pore Size and Catalytic Activity in Cobalt Iron Layered Double Hydroxides and Spinels by Microemulsion-Assisted pH-Controlled Co-Precipitation” ChemSusChem, 2023, 16, e202202015, DOI: 10.1002/cssc.202202015.
R. Aymerich-Armengol, P. Cignoni, P. Ebbinghaus, J. Linnemann, M. Rabe, K. Tschulik, C. Scheu, J. Lim “Mechanism of Coupled Phase/Morphology Transformation of 2D Manganese Oxides through Fe Galvanic Exchange Reaction” J. Mater. Chem. A, 2022, 10, 24190, DOI: 10.1039/D2TA06552E.
W. Xiang, N. Yang, X. Li, J. Linnemann, U. Hagemann, O. Ruediger, M. Heidelmann, T. Falk, M. Aramini, S. DeBeer, M. Muhler, K. Tschulik, T. Li “3D Atomic-Scale Imaging of Mixed Co-Fe Spinel Oxide Nanoparticles during Oxygen Evolution Reaction” Nat. Commun., 2022, 13, 179, DOI: 10.1038/s41467-021-27788-2.
M. Azimzadeh Sani, N. G. Pavlopoulos, S. Pezzotti, A. Serva, P. Cignoni, J. Linnemann, M. Salanne, M.-P. Gaigeot, K. Tschulik “Unexpectedly High Capacitance of the Metal Nanoparticle/Water Interface: Molecular-Level Insights into the Electrical Double Layer” Angew. Chem., Int. Ed., 2022, 61, e202112679, DOI: 10.1002/anie.202112679.
Z. Liu, M. Corva, H. M. A. Amin, N. Blanc, J. Linnemann, K. Tschulik “Single Co3O4 Nanocubes Electrocatalyzing the Oxygen Evolution Reaction: Nano-Impact Insights into Intrinsic Activity and Support Effects” Int. J. Mol. Sci., 2021, 22, 13137, DOI: 10.3390/ijms222313137.
J. Linnemann, K. Kanokanchana, K. Tschulik “Design Strategies for Electrocatalysts from an Electrochemist’s Perspective” ACS Catal., 2021, 11, 9, 5318-5346, DOI: 10.1021/acscatal.0c04118.
Meet the Team
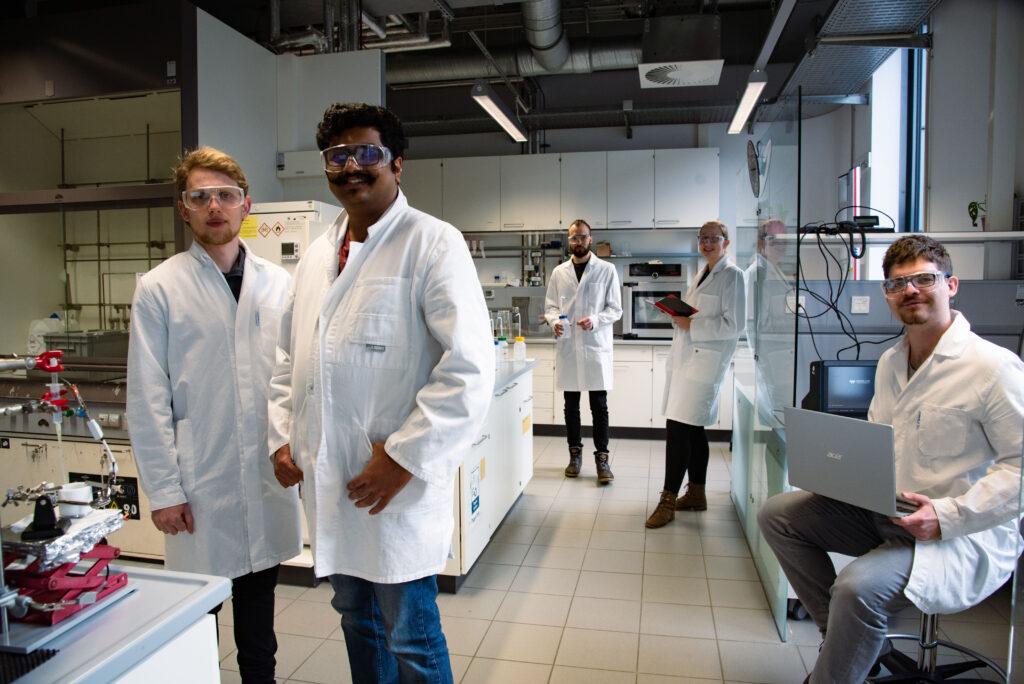
We are happy to welcome Bachelor and Master students for thesis projects, specialization-/in-depth practicals in our lab.
Get in touch
… or visit us at ZEMOS
If you are a curious chemist/material scientist (M.Sc./M.Eng.) or about to become one and interested in joining the project, please contact Julia Linnemann.
Dr. Julia Linnemann
Ruhr University Bochum
Analytical Chemistry II
Universitätsstr. 150, ZEMOS 2.31
D-44801 Bochum
+49 234 32 19076
julia.linnemann@ruhr-uni-bochum.de